WTF is Internet Of Bio Nano Things (IoBNT) and How Secure Is It?
Internet of Bio-Nano Things (IoBNT) is a domain where biochemical processes inside the human body communicate the cyber world of the internet. IoBNT paradigm stems from synthetic biology and nanotechnology tools and enables the engineering of biological embedded computing devices called nano-machines.
Nano-machines are powerful and functional man-made tiny devices. The functionality of these devices is inspired by the behavior of atomic and molecular structures composed of nanoscale components.
They not only function as computers but also establish connections with the environment (human body) to detect a physical quantity, as living organisms.
Communication inside the human body is as old as the existence of mankind. Indeed, the human body is a large-scale heterogeneous communication network of nano-networks as it is composed of billions of interacting nano-machines, i.e., cells, whose functionalities primarily depend on nanoscale molecular communications.
Hence, the vital conditions of the human body directly depend on the performance, reliability, and continuous functioning of intra-body molecular nano-networks.
Architecture of Internet of Bio-Nano Things
​
​
Internet of Bio-Nano Things (Image Source: IEEE Communications Magazine)
IoBNT architecture is an extended version of the Internet of Things paradigm. IoBNT uses the cyber communication backbone of IoT. The main difference is in the perception layer, where the sensors are deployed. The sensors used in IoBNT are nano-scale and use the nano-scale communication technologies (Molecular Communication/ Terahertz based Nano electromagnetic Communication).
Following are the entities involved in IoBNT architecture:
Bio-Nano Things
Bio-Nano Things are biological computing machines that collect sensory data from the environment (inside the human body) and pass it to the bio cyber interface. Bio nano things are used interchangeably with nanomachines.
In order to develop efficient and novel nano-machines and to understand the communication mechanism between nano-machine, a study of biological cell architecture and their interactions has been proved helpful. Bio nano things are deployed in the form of nanonetworks inside the human body for biomedical applications. Below are five components of nanomachines.
​
​
​
​
​
​
​
​
​
​
​
Image Source: FUTURE NOW THE IFTF BLOG
1. Control Unit: It contains the embedded software, which aims to perform the intended task of nanomachine. The control unit controls all the other components and could have a storage unit. The nucleus of a biological cell is responsible for realizing its intended tasks. Similar to software conditional expressions biological control unit encodes protein structures, data units, and regulatory sequences.
2.Communication Unit: The communication mechanism of nanomachine is realized through transceivers. Transceivers allow the embedded system to exchange information by transmitting and receiving messages at the nano level. The inter-cellular communication is realized through the gap junctions, hormonal and pheromonal receptors placed on the membrane of the cell.
3.Reproduction unit: It contains the instructions to fabricate the components of nano-machines and then to replicate them. This process takes place when nanomachines are replicated by saving the code of nanomachines in molecular sequences.
4.Power Unit: Power unit supplies stored energy to all the other components of nano-machines, to maintain the electrical current in embedded software. Mitochondrion, chloroplast, and Adenosien Triphosphate are some of the substances of cells that correspond to the external chemical reactions to produce energy. This chemical energy is stored in the cell reservoirs and supplied to regulate the other components of the cell.
5.Sensor and Actuators: This unit provides an interface between the environment and nanomachine. The sensing and actuation is the ability of a biological cell to distinguish external molecules or stimuli e-g chloroplast of plants and flagellum of bacteria.
​
Bio cyber Interface
​
​
​
​
​
​
​
​
​
​
​
​
​
​
Electronic tattoo can be used as bio cyber interface to communicate with in body biological nanonetwork(Image Source: IEEE Communications Magazine)
Biocyber interface is a specialized micro device that is usually implanted on the outer parts of the body. Bio cyber Interface acts as the transduction unit, which translates biochemical signals from the human body into electrical signals and electrical commands coming from healthcare providers into biochemical signals for in-body communication. Bio cyber interface has wireless communication capability which is used to communicate with gateway devices. The communication frequency of bio cyber interface is kept weak on purpose so that the high-frequency electromagnetic waves do not interfere with normal body functionality.
Gateway devices
​
​
​
​
​
​
​
​
​
​
​
​
​
​
Image Source: Medical News Today
The gateway devices in IoBNT are usually a smartphone, tablets, PDAs or other wireless communication enabled handheld devices. Bio cyber interface communicates with gateway devices to send data and receive commands from the remote healthcare provider.
Internet access point
The connectivity mechanisms to forward and receive messages from healthcare provider/medical server. For example WiFi, 4G/5G, or other wireless communication mechanisms.
Medical Server
​
​
​
​
​
​
​
​
​
​
​
​
​
​
​
Photo by National Cancer Institue on Unsplash
It can be a cloud-based service for storing and processing health-related data of patients and a web portal used by healthcare providers to analyze reports and consign treatment
Communication among Nano-machines
Nano-machines are only able to perform trivial tasks on their own; thus communication among nano-machines is very important to realize more complex tasks . Nano-machine communication technologies are divided into four groups namely:
​
-
Nanoscale Electromagnetic communication
-
Nanoscale Acoustic Communication
-
Nanoscale Mechanical Communication
-
Molecular Communication
Nanoscale Electromagnetic Communication
This type of communication is based on the transmission and reception of electromagnetic waves between novel nano materials such as carbon nanotubes and graphene based nanoribbons. The traditional transceiver of classical wireless communication is not feasible for nano-scale communication, however novel graphene based nano-materials have shown potential to overcome this limitation.Future electromagnetic nanonetworks are envisioned to operate on terahertz band, while few terahertz channel exist to date. Electromagnetic communication can make communication possible from micro device to nano device, and research is being made for the communication between nano devices or from nano to micro device.
Nanoscale Acoustic Communication
Acoustic communication is realized by the transmission of ultrasonic waves through nano machine integrated transducers .These transducers should be capable to sense the variety of pressure and then react accordingly. Currently the size of transducers is the major barrier to implement this communication mechanism at nano-scale.
Nanoscale Mechanical Communication
In nano mechanical communication, the information is sent through nano machines that are linked physically. One of the major drawbacks for this communication technique in nano communication context is physical connection between devices. Therefore it is not workable for the applications where nano-machines have to be placed at distant locations.
​
Molecular Communication
​
​
​
​
​
​
​
​
​
​
​
​
​
Image source: IEEE Xplore
Molecular Communication (MC) is a molecule based communication paradigm that enables transmission of bio-chemical information (e.g. status of living organisms), which is not feasible using traditional communication. Molecules encoded with information to be transmitted, are called information molecules. The information molecules activate bio-chemical reaction at receiver and may recreate phenomena and/or chemical status, which sender then transmits. Molecular communication (MC) is considered the most promising nano networking mechanism due to its nano-sized transceivers that can easily integrate into nano machine. MC is bio-inspired and closely relates to the communication phenomena existent in nature for years.MC is also paramount choice for bio medical applications that involve intra body communication. Different schemes for molecular communication can be categorized into short range communication using calcium signaling, medium range signaling using molecular motors and long range signaling using pheromones.
Security for the Internet of Bio-Nano Things
Nano networking is a novelty in the IoBNT domain. Thus, here we focus on the security requirements in nanonetworking only. The cybersecurity for IoT has already been discussed extensively.
Global Security Goals
Global security goals for the classic communication paradigm are CIA (Confidentiality, Integrity, and Availability). These classic security goals will remain the same even in the context of the nano communication paradigm. For more holistic security requirements we have added ‘Authenticity’ as the fourth security requirement.
Confidentiality
It is the most important issue in network security. This goal ensures that the context of the message exchanged between sender and receiver should not be accessible to an unauthorized entity. Encryption techniques can be used to add confidentiality to the communication.
Integrity
It assures that the received information is complete and correct i.e., without being modified by an external entity. To ensure integrity in communication MAC (Message Authentication Code) hash functions can be used.
Availability
It assures that information is always available; the attacker should not be able to disrupt communication at any time. Setting redundancy to the network can assure availability.
Authenticity
It ensures that the source of message transmission is reliable and stops the attacker from sending fake messages.
Types of Attacks and Threats for IoBNT
Identification of attacks and threats is also important for network security. The attacks can be performed by two types of attackers; Internal attackers and External attackers.
Internal attackers are part of the system and have access to credentials and other information required to communicate with other system entities.
External attackers in the scenario of nano communication can be divided into two types: Local and remote attackers. Local attackers are located in the vicinity of attacked nanosystem. Attacks like eavesdropping and spoofing can be performed by these attackers. Remote attackers have to become local attackers before launching the attack.
The types of attacks launched by these attackers are classified into four groups:
-
Disclosure: These types of threats include access to the system by unauthorized users. In the case of nano-networks, these types of attacks are complex to launch when using mechanical or molecular communication. However electromagnetic and acoustical communication opens the door for the attackers as the covered is area is relatively large.
-
Deception: Attackers can falsify or manipulate data in this type of attack. Injecting false information in the network can challenge the system reliability. Integrity checks can be performed to limit these attacks. However computational protection schemes
-
Disruption: The availability and reliability can be stalled by this kind of attack. Given in the context of nano-networks the attacker can modify the parameters such as temperature and pH level to disrupt communication.
-
Usurpation: Unauthorized entities can control system services or entities, beyond just disrupting the system. This attack can enable attackers to cause malfunctions or even take over the entire system.
Other types of attacks adopted from traditional networks are listed below:
-
Eavesdropping: As the name suggests the attacker snoops the communication between two nodes.
-
Spoofing/Altering/Replaying/Injection: Attackers try to become trustable by spoofing other nodes and broadcast malicious information.
-
Loops: Forwarding of packets to nodes that are not intended for that message in form of a loop.
-
Selective Forwarding: Dropping some of the packets that must be forwarded to the next hop of deciding whether to forward or not.
-
Black Hole: Type of selective forwarding where packets are dropped.
-
Sink Hole: Corrupted node creates an artificial sinkhole by attracting as much traffic as possible.
-
Warm Hole: The routing is disturbed by forwarding messages far way in the network through a secret channel.
-
Sybil Attacks: Geographical routing protocols are disturbed by a malicious node claiming multiple identities.
-
Flooding attack: Availability and energy of potential nodes are overtaken by corrupted nodes through sending fake messages.
-
Desynchronization attack: Sequence numbers of messages sent, are manipulated by the malicious nodes.
-
Jamming attack: The attacker disrupts communication between other nodes by creating noise in the communication channel.
-
Node Capture: Nodes are captured to get cryptography keys and sensors can also be reprogrammed to make them malicious
.
Bio-Inspired Approaches as Security measures for the Internet of Bio-Nano Things
Existing mechanisms of security and privacy used for traditional data communication networks cannot be applied directly to the nano communication network paradigm.
The existing cryptography and encryption techniques such as AES and RSA demand high computational power whereas nano-machines can only handle light-weight security solutions due to their nano-scale capacity. Using classical cryptography might be inefficient if only limited information is transmitted (like sending a small specific molecule to send one bit of information). Then adding a digital signature or long cryptography message authentication code is not appropriate. Many secure localization existing schemes are based on time-of-flight measurements; these are not directly applicable as they would require sub-nanosecond accuracy. However, there exist a number of bio-inspired algorithms for security that can be modified or down-scaled to the nanoscale level to make them feasible for nano-networks. Molecular Communication (MC) is one of the communication mechanisms of nano networking that uses molecules for information exchange. MC is itself bio-inspired and adapts the communication mechanisms existent in nature e-g communication inside the human body. There is an established research area that aims at using Bio-inspired approaches to solve problems according to the application domain. There are three research areas of bio-inspired approaches:
Bio-inspired computing represents a class of solutions that focus on providing efficient computing solutions e-g optimization algorithms and pattern recognition.
Bio-inspired systems class provides nature-inspired solutions for massively distributed and collaborative systems. Bio-inspired networking class provides strategies for efficient and scalable networking under uncertain conditions.
There exists a classification of bio-inspired approaches that provides efficient solutions for security to the computer networks.
Artificial Immune System (AIS)
The artificial immune system has been defined by Castro and Timmis as “adaptive systems, inspired by theoretical immunology and observed immune functions, principals and models, which are applied to problem-solving”. There are a number of appealing features that make an immune system, whether it is a natural or artificial suitable candidate for securing nano applications using molecular communication. Those features are robustness, reinforcement, memory, distributed, adaptive, recognition and dynamically changing coverage.
The main three research fields related to AIS are (1) Immune networks (2) Clonal Selection (3) Negative Selection.
Swarm Molecular Security Approaches
The concept of the swarm is referred to a grouping of individual units to accomplish complex tasks that are difficult to be realized alone. Swarm based security approaches are highly potential for nano-networks security as they can be realized in lightweight systems. This type of security approach is highly effective since they are robust, self-configurable, and adaptive. The mechanisms adopted in nature to defend against attacks and intruders can be treated with swarm intelligence. Similarly, nanorobots have minimal intelligence and capabilities individually, but when they form a group, they can provide an effective mechanism against intruders.
Bio-Chemical cryptography
Falko Dressler et al have defined biochemical cryptography as “a primitive that may be used for efficiently securing biologically based information channels.” The biochemical cryptography techniques proposed so far use DNA molecules for information encryption.
Significance of Security on the Internet of Bio-Nano Things
By connecting the human body to the outside cyber world through IoBNT poses a number of threats and security issues to the human body. There are a number of possible threats and attacks that can be launched to disrupt communication. Ian Akyildiz and his group members have coined the term "Bio Cyber terrorism" for IoBNT attacks in their pioneering work on IoBNT.Security mechanisms used for traditional networks demand high computation power which is beyond the capabilities of nano-machines. The available memory and processing capabilities of nano-machines are extremely limited, which makes use of complex communication algorithms and protocols impractical in nano regime. There is a huge asymmetry between the computational power of desktop computers and a single nano-machine. This limitation might affect the achievable security level, as one might have to work for short key lengths due to resource constraints which would allow attackers to easily perform brute-force attacks using high-performance computing e-g available through graphic cards. Bio-Inspired networking is an established research area that provides solutions, adapted from nature to a number of computing problems. IoBNT has many potential health applications which facilitate patients to relieve from lengthy medical tests, sparing time for appointments from health expert and necessity of being on the same location as that of health provider.
(Featured Image Source: IEEE Globecom)
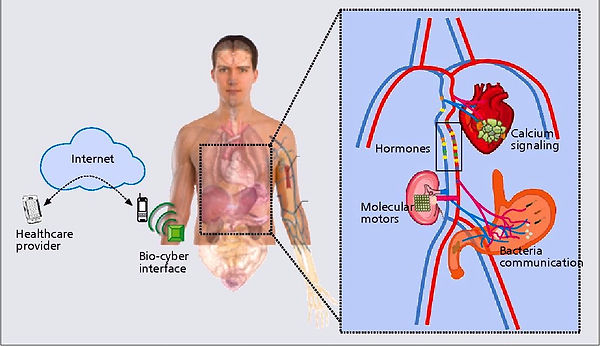
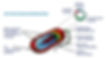


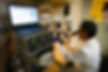
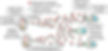
THE INTERNET OF NANO THINGS
ABSTRACT: Nanotechnology promises new solutions for many applications in the biomedical, industrial and military fields as well as in consumer and industrial goods. The interconnection of nanoscale devices with existing communication networks and ultimately the Internet defines a new networking paradigm that is further referred to as the Internet of Nano-Things. Within this context, this paper discusses the state of the art in electromagnetic communication among nanoscale devices. An in-depth view is provided from the communication and information theoretic perspective, by highlighting the major research challenges in terms of channel modeling, information encoding and protocols for nanonetworks and the Internet of Nano-Things.
INTRODUCTION: Nanotechnology is enabling the development of devices in a scale ranging from one to a few hundred nanometers. At this scale, a nanomachine is defined as the most basic functional unit, integrated by nano-components and able to perform simple tasks such as sensing or actuation. Coordination and information sharing among several nanomachines will expand the potential applications of individual devices both in terms of complexity and range of operation [1, 2]. The resulting nanonetworks will be able to cover larger areas, to reach unprecedented locations in a non-invasive way, and to perform additional in-network processing. Moreover, the interconnection of nanoscale devices with classical networks and ultimately the Internet defines a new networking paradigm, to which we further refer as the Internet of Nano-Things.
Despite several papers on nano-devices and their applications are published every year, it is still not clear how nanomachines are going to communicate. For the time being, we envision two main alternatives for communication in the nanoscale, namely, molecular communication and nano-electromagnetic communication: •Molecular communication: this is defined as the transmission and reception of information encoded in molecules [1, 3].
Molecular transceivers are expected to be easily integrated in nano-devices due to their size and domain of operation. These transceivers are able to react to specific molecules, and to release others as a response to an internal command or after performing some type of processing.
•Nano-electromagnetic communication: this is defined as the transmission and reception of electromagnetic (EM) radiation from components based on novel nanomaterials [2, 4]. The unique properties observed in these materials will decide on the specific bandwidth for emission of electromagnetic radiation, the time lag of the emission, or the magnitude of the emitted power for a given input energy.
In this article, we focus on electromagnetic communication among nano-devices and provide an in-depth view of this new networking paradigm from the communication and information theory point of view. We begin our discussion by introducing a reference architecture for the Internet of Nano-Things. We motivate the study of the Terahertz band for nano-electromagnetic communication and outline the main research challenges in terms of channel modeling, information modulation and networking protocols for nano-devices. Finally we conclude the article.
NETWORK ARCHITECTURE: The interconnection of nanomachines with existing communication networks and eventually the Internet requires the development of new network architectures. In Fig. 1, we introduce the architecture for the Internet of Nano-Things in two different applications, namely, intrabody nanonetworks for remote healthcare, and the future interconnected office:
•In intrabody networks, nanomachines such as nanosensors and nanoactuators deployed inside the human body are remotely controlled from the macroscale and over the Internet by an external user such as a healthcare provider. The nanoscale is the natural domain of molecules, proteins, DNA, organelles and the major components of cells. Amongst others, existing biological nanosensors and nanoactuators provide an interface between biological phenomena and electronic nano-devices [2], which can be exploited through this new networking paradigm. •In the interconnected office, every single element normally found in an office and even its internal components are provided of a nanotransceiver which allows them to be permanently connected to the Internet. As a result, a user can keep track of the location and status of all its belongings in an effortless fashion. Convenience and almost seamless deployment demand for tiny and non-obtrusive devices. Amongst others, the possibility to harvest vibrational, mechanical or even EM energy from the environment [5], an ultra-low power consumption and reasonable computing capabilities, motivate the use of new nanomaterials in the development of these devices.

Figure 1.Network architecture for the Internet of Nano-Things: a Intrabody nanonetworks for healthcare applications; b The interconnected office.
Regardless of the final application, we identify the following components in the network architecture of the Internet of Nano-Things:
Nano-nodes: these are the smallest and simplest nanomachines. They are able to perform simple computation, have limited memory, and can only transmit over very short distances, mainly because of their reduced energy and limited communication capabilities. Biological nanosensor nodes inside the human body and nanomachines with communication capabilities integrated in all types of things such as books, keys, or paper folders are good examples of nano-nodes.
Nano-routers: these nano-devices have comparatively larger computational resources than nano-nodes and are suitable for aggregating information coming from limited nanomachines. In addition, nano-routers can also control the behavior of nano-nodes by exchanging very simple control commands (on/off, sleep, read value, etc.). However, this increase in capabilities involves an increase in their size, and this makes their deployment more invasive.
Nano-micro interface devices: these are able to aggregate the information coming from nanorouters, to convey it to the microscale, and vice versa. We think of nano-micro interfaces as hybrid devices able both to communicate in the nanoscale using the aforementioned nanocommunication techniques and to use classical communication paradigms in conventional communication networks. Gateway: this device enables the remote control of the entire system over the Internet. For example, in an intrabody network scenario, an advanced cellphone can forward the information it receives from a nano-micro interface in our wrist to our healthcare provider.
In the interconnected office, a modem-router can provided this functionality. Despite the interconnection of microscale devices, the development of gateways and the network management over the Internet are still open research areas, in the remaining of this article we mainly focus on the communication challenges among nanomachines.
COMMUNICATION CHALLENGES FOR ELECTROMAGNETIC NANO NETWORKS
The Internet of Nano-Things begins at the networking of several nanomachines.
Nanonetworks are not just downscaled networks, but there are several properties stemming from the nanoscale that require us to totally rethink well-established networking concepts. In the following, the main challenges from the communication perspective are discussed in a bottom-up fashion, by starting from the physical nanoscale issues affecting a single nanomachine up to the nanonetworking protocols.
The design flow for the development of nanonetworks is shown in Fig
FREQUENCY BAND OF OPERATION OF ELECTROMAGNETIC NANO-TRANSCEIVERS
The communication opportunities and challenges at the nanoscale are strongly determined by the frequency band of operation of future nano-transceivers and specially nano-antennas. Currently, graphene-based nano-antennas have been proposed for nanoscale communications [6, 7]. These antennas are not just mere reductions of classical antennas. Indeed, the wave propagation velocity in graphene can be up to one hundred times below the speed of light in vacuum. As a result, the resonant frequency of nanoantennas based on graphene can be up to two
very high-power source is needed to excite the CNT. Because of this, it does not seem technologically feasible to efficiently radiate above a few micrometers by using a mechanically resonating CNT and, thus, we envision future electromagnetic nanonetworks to operate in the Terahertz band. Nonetheless, we can still use the CNT-based nano-mechanical receiver to control the nanomachines from the macro- and microscale. For example, a conventional AM/FM transmitter can be used to simultaneously activate or deactivate thousands of nano-devices. Focusing on the Terahertz band, we should emphasize that while the frequency regions immediately below and above this band (the microwaves and the far infrared, respectively) have been extensively investigated, this is one of the least-explored frequency zones in the EM spectrum. Therefore, the first research challenge for electromagnetic nanonetworks is to develop new channel models for the Terahertz band.
CHANNEL MODELING: Thinking of the applications of nanonetworks within the Internet of Nano-Things paradigm, there is a need to understand and model the Terahertz channel in the very short range, i.e., for distances much below 1 meter. In [11] we investigated the properties of the Terahertz band in terms of path-loss, noise, bandwidth and channel capacity which we are presenting briefly next. Path-loss— The total path-loss for a traveling wave in the Terahertz band is contributed by the spreading loss and the molecular absorption loss. The spreading loss accounts for the attenuation due to the expansion of the wave as it propagates through the medium, and it depends only on the signal frequency and the transmission distance. The absorption loss accounts for the attenuation that a propagating wave will suffer because of molecular absorption. This phenomenon depends on the concentration and the particular mixture of molecules encountered along the path. Different types of molecules have different resonant frequencies and, in addition, the absorption at each resonance is not confined to a single center frequency, but spread over a range of frequencies. As a result, the Terahertz channel is very frequency-selective. In addition to this, scattering from nano-particles and multi-path propagation can affect the signal strength at the receiver. Noise— The ambient noise in the Terahertz channel is mainly contributed by the molecular noise. Molecular absorption does not only attenuate the transmitted signal, but it also introduces noise. The equivalent noise temperature at the receiver is mainly determined by the number and the particular mixture of molecules found along the path and the transmission distance. The molecular noise is not white but colored. Indeed, because of the different resonant frequencies of each type of molecules, the power spectral density of noise has several peaks. Moreover, this type of noise only appears when transmitting, i.e., there will be no noise unless the channel is being used.
orders of magnitude below that of nano-antennas built with non-carbon materials. In particular, in [8] we determined that a 1 μm long graphene-based nano-antenna based either on a graphene nanoribbon (GNR) or carbon nanotube (CNT) can only efficiently radiate in the Terahertz range. Interestingly enough, this matches the initial predictions for the frequency of operation of graphene-based RF transistors [8]. Alternatively, it has been shown that it is possible to receive and demodulate an electromagnetic wave by using a single carbon nanotube that mechanically resonates at the wave frequency [9]. In this case, the mechanical antenna is integrated by a CNT which has one of its ends connected to a very high voltage source and the other end is left floating. When the nanotube is irradiated by an EM wave, the electrons at the free tip vibrate. If the frequency of the EM wave matches the natural resonant frequency of the CNT, these vibrations become significant and the nanotube is able to demodulate the incoming signal. For example, a 1 μm long nanotube can mechanically resonate at frequencies around a few hundreds of Megahertz. The use of EM waves in the Megahertz range can initially be more appealing than the radiation in the Terahertz band, provided that by transmitting at lower frequencies, nanomachines could communicate over longer distances. However, the energy efficiency of the process to mechanically generate EM waves in a nanodevice is predictably very low [10]. In addition, a
